A Promising Energy Solution for the Future
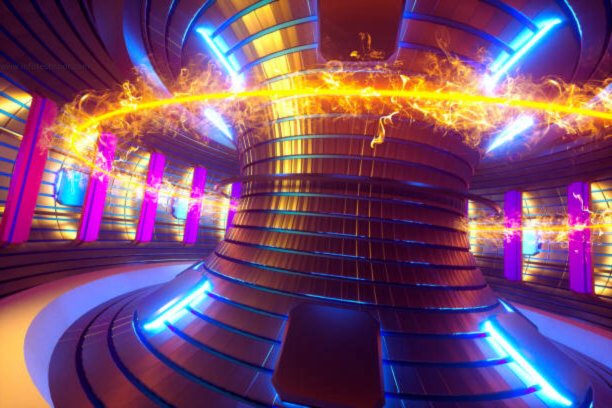
An Introduction
It is a form of power generation that uses nuclear fusion reactions to produce electricity. Nuclear fusion is a process in which two lighter atomic nuclei combine to form a heavier nucleus while releasing energy. Fusion technology aims to harness this energy in devices called fusion reactors.
Why Fusion Technology?
It has many potential advantages over other forms of power generation, such as fossil fuels, nuclear fission, or renewable sources. Some of these advantages are:
- Abundant and clean fuel: It uses hydrogen isotopes. Deuterium can be extracted from seawater, while tritium can be produced from lithium, which is also abundant on Earth. Unlike fossil fuels, fusion fuel does not produce greenhouse gases or air pollutants. Unlike nuclear fission, fusion fuel does not produce long-lived radioactive waste or pose the risk of nuclear proliferation or meltdown.
- High energy density: It can produce a large amount of energy from a small amount of fuel. For example, one gram of deuterium-tritium fuel can produce the same amount of energy as 10 tons of coal or 50 barrels of oil.
- Safe and reliable operation: It does not rely on chain reactions or critical mass, unlike nuclear fission. This means that the fusion reaction can be controlled and stopped easily by adjusting the external conditions. Fusion reactors also have passive safety features, such as magnetic confinement and low fuel inventory, that prevent any catastrophic accidents or explosions.
How Fusion Technology Works?
It works by creating plasma, which is a state of matter where atoms are ionized and separated into electrons and nuclei. The plasma is heated and compressed to reach the conditions needed for fusion reactions to occur. The most common fusion reaction is between deuterium and tritium, which produces helium and a neutron, along with a large amount of energy.
Reactants |
Products |
Energy Released |
Deuterium + Tritium |
Helium + Neutron |
17.6 MeV |
The energy released by the fusion reaction can be converted into electricity in two ways:
- Direct conversion: The charged particles (helium and electrons) can be captured by electric fields and converted into electric current directly. This method has high efficiency but requires advanced technology and materials.
- Indirect conversion: The neutrons can be absorbed by a blanket material surrounding the plasma, which heats up and transfers the heat to a conventional steam turbine generator. This method has a lower efficiency but is more feasible with existing technology and materials.
What are the Challenges of Fusion Technology?
It faces many scientific and engineering challenges before it can become a viable source of power generation. Some of these challenges are:
- Reaching the Lawson criterion: The Lawson criterion is a measure of the minimum product of plasma density, temperature, and confinement time needed to achieve net power from fusion reactions. The current leading designs for fusion reactors are the tokamak and the inertial confinement fusion (ICF) by laser, which aims to reach the Lawson criterion by different methods of heating and confining the plasma.
- Managing the neutrons: The neutrons produced by the fusion reaction are energetic and can damage the reactor materials over time. The neutrons also carry most of the energy released by the fusion reaction, which needs to be captured efficiently by the blanket material. The blanket material also needs to breed more tritium from lithium to sustain the fusion fuel cycle.
- Developing suitable materials: The materials used for fusion reactors need to withstand high temperatures, pressures, radiation, and magnetic fields. They also need to have low activation, high thermal conductivity, and good mechanical properties. Some of the materials being developed for fusion reactors are beryllium, tungsten, steel alloys, ceramics, and superconductors.
What is the Status of Fusion Technology?
It is still in the experimental stage of development, with no device yet achieving net power output. However, there have been significant advances and breakthroughs in recent years that demonstrate the feasibility and progress of it. Some of these achievements are:
- JET: The Joint European Torus (JET) is the largest and most powerful tokamak in operation. It achieved a world record of 16 megawatts of fusion power output in 1997, with an input power of 24 megawatts1. In 2022, it has doubled its record by producing 59 megajoules of energy over five seconds (11 megawatts of power), with an input power of 32 megawatts2. JET is also the first device to use a beryllium-tungsten wall, which is similar to the material planned for ITER.
- ITER: The International Thermonuclear Experimental Reactor (ITER) is a multinational project to build the world’s largest and most advanced tokamak. It aims to produce 500 megawatts of fusion power output with an input power of 50 megawatts, for at least 400 seconds3. ITER is expected to start its first plasma experiments in 2025 and achieve its full fusion power by 2035.
- NIF: The National Ignition Facility (NIF) is the world’s largest and most energetic laser system. It uses 192 laser beams to compress and heat a tiny capsule of deuterium-tritium fuel to create fusion reactions. It aims to achieve ignition, which is the point where the fusion reactions produce more energy than the energy input by the laser. In 2021, NIF achieved a record of 1.35 megajoules of energy output with an input energy of 1.9 megajoules, which is close to the ignition threshold.
Conclusion
It is a promising form of power generation that can provide abundant, clean, and safe energy for the future. However, it also faces many challenges that require further research and development. It is moving from being a physics problem to an engineering one, as more experiments and projects are being conducted to demonstrate its feasibility and performance. It is not yet ready for commercial use, but it is getting closer every day.